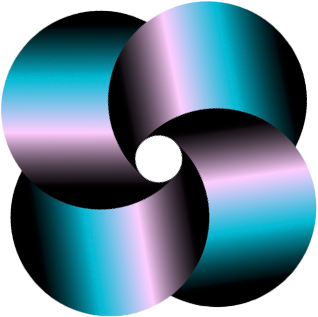
AlphaPerrin Computational™
Design groundbreaking molecules.
Accelerate your research. Risk-free.
AlphaPerrin’s computational chemistry platform empowers you to streamline In Silico design and development. For a limited time, unlock the full potential of AlphaPerrin with our exclusive 30-Day Free Trial
-
-
-
Full Access to AlphaPerrin’s powerful suite of tools for molecule design, simulation, and property prediction.
-
Unrestricted use of our extensive database of building blocks and materials.
-
Seamless Integration with your existing workflows.
-
Expert Support from our team of computational chemistry specialists (limited consultations may apply).
-
-
Benefits of AlphaPerrin for In Silico Design:
-
-
-
-
Faster Development Cycles: Iterate on molecule designs quickly and efficiently.
-
Reduced Costs: Minimize reliance on expensive lab experiments.
-
Enhanced Accuracy: Predict properties with exceptional precision.
-
Improved Success Rates: Increase the likelihood of identifying optimal candidates.
-
-
-
Ready to Take Your In Silico Design To The Next Level?
Computational Chemistry for Molecular and Drug Design.
Personal In Silico Labs.
Secure Cloud Access.
Six compelling reasons to choose AlphaPerrin for your drug development needs:
Cost Efficiency
Computational chemistry and in silico labs significantly reduce the costs associated with reagents, materials, and physical lab space required for wet labs.
Speed To Market
Simulations and computational models can rapidly screen and optimize drug candidates, accelerating the initial phases of drug development compared to the slower pace of wet lab experiments.
Safety
In silico methods eliminate the risks associated with handling hazardous chemicals and conducting potentially dangerous experiments, ensuring a safer research environment.
Scalability
Computational models can easily be scaled to study larger and more complex molecular systems, which may be challenging or impractical in wet labs.
Predictive Power
Advanced algorithms and simulations provide detailed insights into molecular interactions and predict properties and behaviors that may not be easily observable in wet lab experiments.
Resource Conservation
In silico labs conserve valuable resources, including rare or expensive materials, by allowing extensive testing and optimization in a virtual environment before any physical trials.
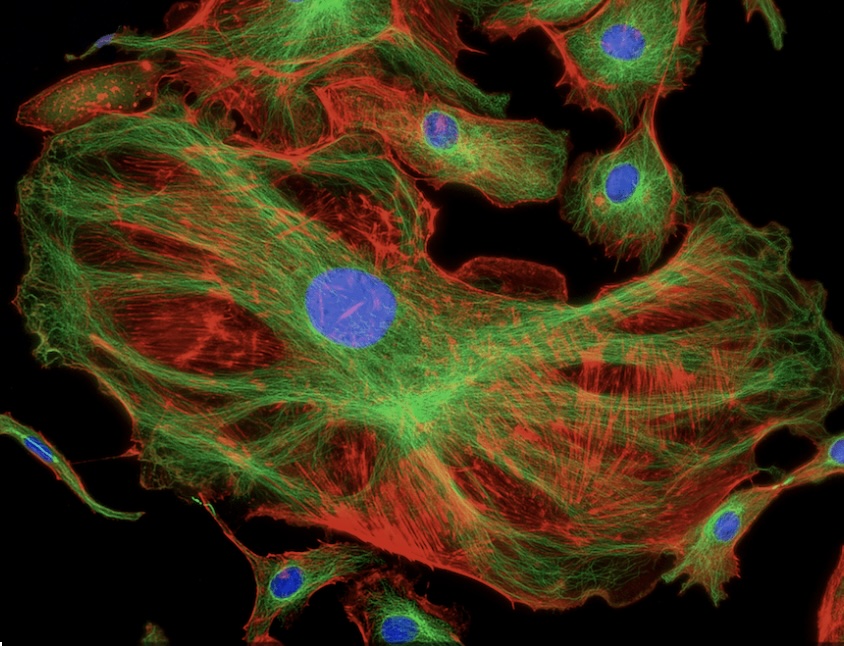
Fundamental Capabilities You Will Have In Your Environment
Starting from the fundamental laws of quantum mechanics, Your Gaussian 16 environment will allow you to predict the energies, molecular structures, vibrational frequencies and molecular properties of compounds and reactions in a wide variety of chemical environments. Gaussian 16’s models can be applied to both stable species and compounds which are difficult or impossible to observe experimentally, whether due to their nature (e.g., toxicity, combustibility, radioactivity) or their inherent fleeting nature (e.g., short-lived intermediates and transition structures).
With Gaussian 16, you can thoroughly investigate the chemical problems that interest you. For example, not only can you minimize molecular structures rapidly and reliably, you can also predict the structures of transition states, and verify that the predicted stationary points are in fact minima or transition structure (as appropriate). You can go on to compute the reaction path by following the intrinsic reaction coordinate (IRC) and determine which reactants and products are connected by a given transition structure. Once you have a complete picture of the potential energy surface, reaction energies and barriers can be accurately predicted. You can also predict a wide variety of chemical properties.
Gaussian 16 offers a wide range of methods for modeling compounds and chemical processes, including:
Molecular Properties
Antiferromagnetic coupling
Atomic charges
ΔG of solvation
Dipole moment
Electron affinities
Electron density
Electronic circular dichroism (ECD)
Electrostatic potential
Electrostatic potential-derived charges
Electronic transition band shape
High accuracy energies
Hyperfine coupling constants (anisotropic)
Hyperfine spectra tensors (including g tensors)
Ionization potentials
IR and Raman spectra*
Pre-resonance Raman spectra*
Resonance Raman spectra
Molecular orbitals
Multipole moments
NMR shielding and chemical shifts
NMR spin-spin coupling constants
Optical rotations (ORD)
Polarizabilities/hyperpolarizabilities
Raman optical activity (ROA)*
Thermochemical analysis
UV/Visible spectra
Vibration-rotation coupling
Vibrational circular dichroism (VCD)*
Vibronic (absorption and emission) spectra
*Harmonic approx. and including anharmonic effects
Fundamental Capabilities
- Molecular mechanicsEGF: Amber, UFF, Dreiding
- Semi-empirical methodsEGF†: AM1, PM6, PM7, DFTB, among others
- Hartree-FockEGF
- Density functional (DFT) methodsEGF, with support for a plethora of published functionals; long-range and empirical dispersion corrections are available where defined
- Complete active space self-consistent field (CASSCF)EGF, including RAS support and conical intersection optimizations.
- Møller-Plesset perturbation theory: MP2EGF, MP3EG, MP4(SDQ)EG, MP4(SDTQ)E, MP5E
- Coupled cluster: CCDEG, CCSDEG, CCSD(T)E
- Brueckner doubles: BDEG, BD(T)E
- Outer Valence Green’s Function (OVGF): ionization potentials and electron affinities
- High accuracy energy models: G1-G4, CBS series and W1 series, all with variants
- Excited state methods: TD-DFTEGF, EOM-CCSDEG and SAC-CIEG
EEnergies; GAnalytic gradients; FAnalytic frequencies; F†Reimplemented with analytic frequencies.
A wide range of Gaussian results can be examined with GaussView’s visualization capabilities:
- Molecule annotations and/or property-specific coloring: e.g., atomic charges, bond orders, NMR chemical shifts
- Plots, including NMR, vibrational and vibronic spectra
- Surfaces or contours: e.g., molecular orbitals, electron density, spin density. Properties such as the electrostatic potential can be visualized as a colorized density surface.
- Animations: e.g., normal modes, IRC paths, geometry optimizations
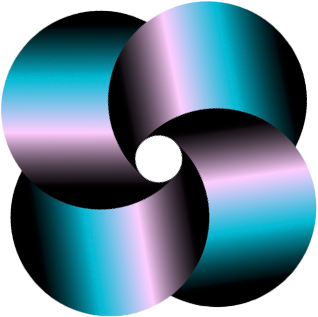